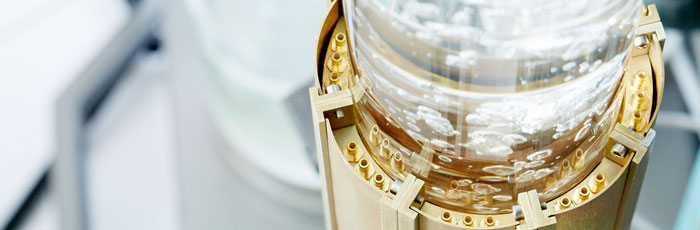
Fluid Mechanics
Focus of our research
Led by Professor Alban Potherat, the Fluid Mechanics group gathers mathematicians and experimentalists to tackle fundamental problems in fluid mechanics arising in natural and industrial processes.
The group's expertise lies in stability theory, turbulence, convection, magnetohydrodynamics, plasmas, particulate and free surface flows, as well as advanced numerical methods. A large part of our research is concerned with astro- and geophysical flows (in particular in planetary interiors), metallurgical and nuclear engineering, and flow measurement.
The research carried out by our members is supported by partners including the Royal Society, the Leverhulme Trust, and EPSRC, as well as a number of industrial partners.
If you wish to find out more about our research, please contact Professor Alban Potherat.
Projects
Feature Project: Transition to Turbulence in Complex Fluids
Pumping fluids through pipes is an important part of many industrial processes. Turbulence during pumping greatly reduces its efficiency. In theory avoiding turbulence is achievable as a disturbance over a certain minimum size is needed to trigger it. Knowledge of this minimum size tells us how smooth the pipe needs to be in order to avoid turbulence. However, it has recently been shown with classical fluids, such as oil and water, that this minimum size is very low indeed – so low that in practice it may be entirely impossible to avoid turbulence for anything above moderate flow rates.
A potential solution would be to change the characteristics of the observed turbulence. Adding small quantities of polymers to a fluid can change its fundamental properties. In this way it is possible to create a shear thinning fluid – one which becomes less viscous as it is forced through a pipe. Although for classical fluids reducing the viscosity actually increases the chances of turbulence, shear thinning fluids exhibit two types of turbulence. Along with the problematic turbulence observed for water or oil, there is also an intermediate ‘weak turbulence’ that has a much smaller impact on pumping efficiency. This proposal seeks to explore and understand the ‘weak state’ and to find conditions for holding off turbulence to improve pumping efficiency.